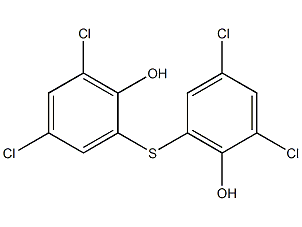
Structural formula
Business number |
02BX |
Molecular formula |
C12H6Cl4O2S |
Molecular weight |
356.05 |
label |
thiochlorophenol,
Thiobisdichlorophenol,
Beding,
Schlorophenol,
Sulfur double-dichlorophenol,
Other small,
antibacterial agent
|
Numbering system
CAS number:97-18-7
MDL number:MFCD00055727
EINECS number:202-565-0
RTECS number:SN0525000
BRN number:2003535
PubChem number:24900609
Physical property data
1. Properties: white crystalline powder.
2. Relative density (g/mL, 20?): 1.73
3. Relative vapor density (g/mL, air=1): Undetermined
4. Melting point (ºC): 185.5?186.5
5. Boiling point (ºC, normal pressure): Undetermined p>
6. Boiling point (ºC, kPa): Undetermined
7. Refractive index: Undetermined
8. Flash point (ºC): Undetermined
9. Specific optical rotation (º): Undetermined
10. Autoignition point or ignition temperature (ºC): Undetermined
11. Vapor pressure (mmHg, 20.2ºC): Undetermined
12. Saturated vapor pressure (kPa, ºC): Undetermined
13. Heat of combustion (KJ/mol): Undetermined
14. Critical temperature (ºC): Undetermined
15. Critical pressure (KPa): Undetermined
16. Log value of oil-water (octanol/water) partition coefficient : Undetermined
17. Explosion upper limit (%, V/V): Undetermined
18. Explosion lower limit (%, V/V): Undetermined
19. Solubility: Insoluble in water, easily soluble in ethanol, acetone, ether, etc.
Toxicological data
1. Acute toxicity: Rat oral LD50:7mg/kg; Orally administered to miceLD50:760mg/kg; Mouse peritoneal cavityLD50?100mg/kg; Mice were intravenously injected with LD50: 18 m g/kg;2. Chronic toxicity/Carcinogenicity: oral administration in miceTDLo?12mg/kg/78W-I;
Ecological data
None
Molecular structure data
Molecular property data:
1. Molar refractive index: 81.84
2. Molar volume (cm3/mol): 202.7
3. Isotonic specific volume (90.2K): 608.8
4. Surface tension (dyne/cm): 81.3
5. Dielectric constant:
6. Dipole moment (10-24cm3):
7. Polarizability: 32.44
Compute chemical data
1. Reference value for hydrophobic parameter calculation (XlogP): 5.8
2. Number of hydrogen bond donors: 2
3. Number of hydrogen bond acceptors: 3
4. Number of rotatable chemical bonds: 2
5. Number of tautomers: 5
6. Topological molecule polar surface area 65.8
7. Number of heavy atoms: 19
8. Surface charge: 0
9. Complexity: 282
10. Number of isotope atoms: 0
11. Determine the number of atomic stereocenters: 0
12. Uncertain number of atomic stereocenters: 0
13. Determine the number of chemical bond stereocenters: 0
14. Number of uncertain chemical bond stereocenters: 0
15. Number of covalent bond units: 1
Properties and stability
White crystalline powder, easily soluble in ethanol, acetone, soluble in dilute alkali solution, insoluble in water.
This product has low toxicity and almost no irritation to the skin.
Storage method
None
Synthesis method
1. Using phenol as raw material, it is first chlorinated to generate 2,4-dichlorophenol, and then sulfurized with sulfur monochloride to generate sulfur bisdichlorophenol. (1) Chlorination: 2,4-dichlorophenol is produced by reacting phenol with chlorine: First, melt the phenol and pass dry chlorine gas at 57-63°C for 35 hours. After the reaction is completed, 2,4-dichlorophenol is obtained. The yield can reach about 91.5%.
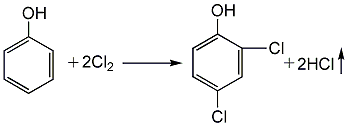
(2) Sulfided dichlorophenol React with sulfur monochloride in the presence of trichlorethylene and anhydrous aluminum trichloride to obtain sulfur bisdichlorophenol: add dichlorophenol, trichlorethylene, and anhydrous aluminum trichloride in the tank in sequence at 50 Add sulfur monochloride at ±2°C, complete the addition in about 2.5 hours, and then react at a constant temperature for 2 hours. Filter, wash with trichlorethylene and dry to obtain crude sulfur bisdichlorophenol. The yield can reach about 51.3%.
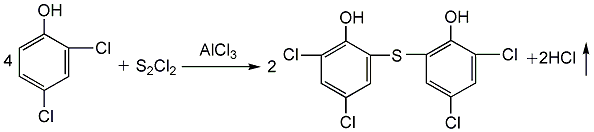
(3) Refined reaction Add crude sulfur bisdichlorophenol into the tank, then add sodium hydroxide solution, heat to dissolve, then cool to 0°C, add sodium chloride, stir to salt out, add saturated sodium chloride solution, and stir evenly. Leave to stand, filter, wash with saturated sodium chloride solution, and recover to obtain sodium thiobisdichlorophenate. Then dissolve it in distilled water, filter it, stir and add 3.5% hydrochloric acid at about 80°C to precipitate, let it stand, and separate the supernatant liquid. The precipitate is washed with distilled water until the pH value is 2, filtered, washed with water until the pH value is about 7, and dried to obtain thiobisdichlorophenol.
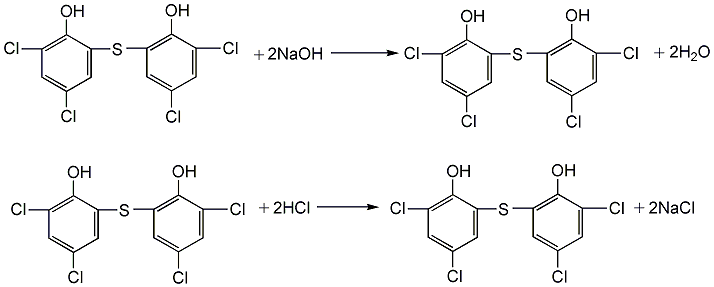
2. Using phenol as raw material Chlorination and sulfurization yield dichlorophenol sulfate.
Dry chlorine gas was passed into the molten phenol (57-63°C). After 35 hours, the yield of 2,4-dichlorophenol reached 91.5%. Then add dichlorophenol, trichlorethylene, and anhydrous aluminum trichloride to the reaction kettle, pass sulfur dichloride (SOCl2) at 48-52°C for 2.5 hours, and react at a constant temperature for 2 hours. After cooling, filter, wash with trichlorethylene, and dry to obtain crude thiochlorophenol. Dissolve crude thiochlorophenol in hot sodium hydroxide solution, add salt to salt out after cooling, and wash with saturated brine to obtain sodium thiochlorophenol. Sodium thiochlorophenol is dissolved in water, filtered and precipitated by adding dilute hydrochloric acid. After standing, filter out the precipitate, wash with water until neutral, and dry to obtain the finished product.
Purpose
1. This product is used as an antibacterial agent and a preservative for natural latex. It also has significant anti-coagulation effects. It can be used alone or in combination with ammonia. The general dosage is more than 0.001%. Latex can be added through emulsification or dispersion. Latex can also be added in the form of alkali metal or ammonium salts. This product is also used in the manufacture of anthelmintics and has a significant killing effect on paragonimiasis metacercariae. It is effective against Fasciolopsis and Clonorchis sinensis.
2.It is an antibacterial agent and veterinary drug. It can be used as a preservative for natural latex. It can be added to the latex through emulsification or dispersion. It can also be added to the latex in the form of alkali metal or ammonium salts. It also has a significant anti-coagulation effect. It can be used alone or It can be used together with ammonia, and the general dosage is more than 0.001%. It is effective in killing or treating paragonimiasis metacercariae, Fasciolopsis sinensis, and the tapeworm S. solium.
extended-reading:https://www.newtopchem.com/archives/39970extended-reading:https://www.bdmaee.net/wp-content/uploads/2022/08/115-9.jpgextended-reading:https://www.newtopchem.com/archives/category/products/page/102extended-reading:https://www.bdmaee.net/wp-content/uploads/2022/08/102-5.jpgextended-reading:https://www.bdmaee.net/spraying-composite-amine-catalyst-nt-cat-pt1003-pt1003/extended-reading:https://www.cyclohexylamine.net/sponge-hardener/extended-reading:https://www.bdmaee.net/wp-content/uploads/2022/08/-NE1060-catalyst–NE1060-foam-catalyst–NE1060.pdfextended-reading:https://www.bdmaee.net/fascat-4210-catalyst/extended-reading:https://www.bdmaee.net/dabco-eg-33-triethylenediamine-in-eg-solution-pc-cat-td-33eg/extended-reading:https://www.bdmaee.net/cas-68928-76-7/